Y.-Q. Zeng1, Y.-J. Wang2, X.-F. Zhou1,3
1. Key Laboratory of Stem Cells and Regenerative Medicine, Institute of Molecular and Clinical Medicine, Kunming Medical University, Kunming, China; 2. Department of Neurology and Centre for Clinical Neuroscience, Daping Hospital, Third Military Medical University, Chongqing, China; 3. School of Pharmacy and Medical Sciences, Sansom Institute, University of South Australia, Adelaide, South Australia, Australia
Corresponding Author: Y.-Q. Zeng, Key Laboratory of Stem Cells and Regenerative Medicine, Institute of Molecular and Clinical Medicine, Kunming Medical University, Kunming, ChinaYue-Qin Zeng, email: z_yueqin@foxmail.com
Abstract
Abstract
The extracellular accumulation of amyloid beta protein (Aβ), reactive gliosis and cerebral amyloid angiopathy (CAA) play critical roles in the pathogenesis of Alzheimer’s disease (AD). Ginkgetin, a biflavone isolated from Ginkgo biloba leaves, was previously reported to exhibit strong neuroprotection against cytotoxic insults induced by oxidative stress and amyloid beta, but it remains unclear whether ginkgetin has therapeutic effect on Alzheimer’s disease (AD) in vivo. In the present study, we investigated 9 months treatment effects of ginkgetin diet in APP/PS1 mice. Our results show that ginkgetin can significantly reduce plasma Aβ levels 59% and Aβ plaque 51% in the brain of APP/PS1 transgenic mice (P<0.05), effectively inhibits cerebral microhemorrhage 69% (P<0.05), significantly decreases astrogliosis 50% and ameliorate inflammation (P<0.05), exhibits several biological properties for AD.
Key words: Ginkgetin, Alzheimer’s disease, inflammation, Aβ, APP/PS1 transgenic mice
Introduction
Alzheimer’s disease (AD) is a chronic neurological disorder characterized by memory deficits, cognitive impairment, and personality changes accompanied by specific structural abnormalities, such as degeneration of neurons and synapses, formation of senile plaques and neurofibrillary tangles (NFT) in the microscopic structures of brain(1). The extracellular accumulation of amyloid-beta (Aβ) peptides as plaques in the brains of AD and the production of intracellular neurofibrillary tangles are major pathophysiological hall marks of this disease (2). Many treatment strategies are based on the Aβ hypothesis, which involve reduction of Aβ generation, inhibition of Aβ aggregation, enhancement of Aβ clearance, interruption of tau hyperphosphorylation and use of more efficacious anti-oxidant or anti-inflammatory agents to delay or prevent disease progression.
G. biloba leaves are widely used as herbal medicine in asthma treatment, coughing, bronchitis and tuberculosis in China (3). G. biloba extract (EGb761H- TanakanH) is one of the most commonly used neutraceuticals marketed in Europe and used for various problems relating to mental health and wellbeing (4). G. biloba extract exerts beneficial effects on memory impairment, stroke, edema, inflammation, Alzheimer’s disease and vascular dementia (5). Various chemical constituents have been isolated from G. biloba leaves, but the two main pharmacologically active groups of compounds present in the G. biloba leave extract are flavonoids and terpenoids (6).
Ginkgetin is the 7, 4’-dimethyl ether derivative of amentoflavone isolated from G. biloba leaves, it consists of a dimer of flavonoids linked to each other by C–C covalent bond and displays many biological and pharmacological activities, including anti-inflammatory, anti-influenza virus, anti-fungal activity, osteoblast differentiation stimulating activity and neuroprotective effects (7), recently, the growing lines of evidence have been accumulated on neuroprotection of ginkgetin, such as protected SH-SY5Y and PC12 cells against atrazine-induced neurotoxicity, exerted strong neuroprotection against cytotoxic insults induced by oxidative stress and amyloid β (8), an positive effects on brain activity and short-term memory in animals and humans suffering from cognitive impairment (9). Although many trials indicate G. Biloba can modestly improve cognitive ability, some inconsistent results from recent trials (10). Overall, there is no clear conclusion that G. Biloba is efficacious for AD.
The aim of this study was to assess the efficacy of ginkgetin in APP/PS1 transgenic mice. Meanwhile, curcumin was used as a positive control because it can effectively disaggregate Aβ and prevent fibril and oligomer formation (11).
Materials and Metholds
Animals
APPSwe/PS1dE9 transgenic mice were obtained from Jackson Laboratory, USA. The APdE9mice were created by coinjection of chimeric mouse/human APPswe and human PS1dE9 (deletion of exon 9) vectors controlled by independent mouse prion protein promoter elements. The two transgenes cointegrated and cosegregate as a singlelocus (12). The line was originally maintained in a C3HeJxC57BL/6J hybrid background. Genotyping of mice was determined by PCR following supplier’s instructions. Mice were given ad libitum access to food and water and kept in a regulated environment (22±2ºC) under a 12h light/dark cycle. Before experiments, all animals were fed with commercial standard diet pellets.
Dietary Intervention
At 3-month-old prior to the onset of AD-type neuropathology, these mice were randomly assigned to four diet groups: ginkgetin group (6 males and 6 females, fed with ginkgetin diet), curcumin group (6 males and 6 females, fed with curcumin diet) and normal diet group (6 males and 6 females, fed with normal diet). Another age and sex matched wild-type littermates (6 males and 6 females, fed with normal diet) were used as a control group. The mean daily food consumption of the mice was 0.08– 0.12 g/g body weight, the corresponding daily ginkgetin [Fig1] (>95% purity by HPLC, Waterstone Pharmaceutical Co., Ltd, Beijing, China) and curcumin (>95% purity by HPLC, Shanghai Tauto Biotech Co., Ltd, Shanghai, China) were about 200 and 80mg/kg/day based on previous report indicating lack of toxicity (13,14), the equivalent consumption in a 60 kg human is about 0.91g/day for ginkgetin and 0.35g/day for curcuminas derived using FDA criteria for converting drug equivalent dosages across species, based on body surface area: human equivalent dose in mg/kg = animal dose in mg/kg x (animal weight in kg/human weight in kg) 0.33(15). The experimental diets were prepared by Zoopery Centre, Kunming Medical University. Control diet was the standard commercial diet purchased from Beijing Keao Xieli Feed Company. All animals were fed with the above diets for 9 months. Food consumption and body weight data were measured every 3 month during treatment period.
All experiments were performed in accordance with the European Communities Council Directive (2010/63/UE) and National Institute of Health Guidelines for the Care and Use of Laboratory Animals, The study had been approved by the Committee for Animal Experiments and Ethics at the Kunming Medical University.
Tissue Sampling and Preparation
At the end of the experiment, mice were sacrificed and blood samples were collected from the right atrium of the heart. The mice were perfused with 50 mL 0.9% saline and the brains were rapidly dissected on the ice. Left brain hemisphere was fixed in 4% paraformaldehyde (pH 7.4) for 24h and incubated another 24h in 30% sucrose for subsequent cryoprotection. Coronal sections of the brain were cut at 35μm thickness with a cryosectioning microtome and stored at 4°C in PBS containing 0.1% sodium azide until use. Right brain hemisphere was snap frozen in liquid nitrogen and stored at -80°C for future biochemical analysis.
AD-type Pathology and Quantitative Image Analysis
The left brain hemisphere was processed according to the protocols described previously (16). Briefly, a series of five equally spaced tissue sections (~1.3mm apart) spanning the entire brain were randomly selected and stained using free-floating immunohistochemistry for total Aβ (Biotin-conjugated mouse anti-Aβ antibody 6E10, Serotec; 1:1000 dilution), activated microglia (rat monoclonal anti-CD45, 1:1000 dilution, Millipore Bioscience Research Reagents, CA, USA), and astrocyte (rabbit polyclonal anti-glial fibrilliary acidic protein, 1:1000 dilution, Dako, Glostrup, Denmark), respectively. Sections were incubated overnight with primary antibodies at 4°C and the reaction products were visualized with diaminobenzidine (No.AB500-500 Slide Kit, Chemical International, Inc. Millipore).
The region of neocortex and hippocampus manually were selected for quantification of total Aβ plaques, microgliosis and astrogliosis. Slides were photographed using a digital microscope colour camera (Olympuse DP73). All images acquired in the same region of neocortex and hippocampus. The images were collected at 4x magnification using constant bulb temperature and exposure. Data are reported as percentage of immune labeled area captured (positive staining) divided by the full area captured. The average of the individual measurements was used to calculate group means and standard errors.
A series of six equally spaced tissue sections (*1mm apart) spanning the entire brain were mounted and stained with Prussian blue working solution (equal parts of freshly made 5% potassium ferrocyanide and 5% hydrochloric acid) for 30 min at room temperature, washed in deionized water, and counterstained with Nuclear fast red. Microhaemorrhage events in the form of the number of Prussian blue-positive profiles in the brains of mouse were counted by two independent observers, and the average number of hemosiderin deposits was calculated per each brain hemisphere.
Biochemical Assay
Quantification of Aβ peptide Levels in the Mouse Brain and Plasma
ELISA analysis of the brain Aβ was processed as described previously (17). Briefly, frozen brain was homogenized and sonicated in TBS containing protease inhibitors. Homogenates were centrifuged at 100,000 xg for 1h at 4°C, and the resultant supernatant was collected, representing the TBS-soluble fraction (Aβ-TBS). The resultant pellet was suspended and sonicated in water containing 2% SDS and protease inhibitors. The SDS solubilized homogenates were centrifuged at 100,000 xg for 1h at 4°C, and the resultant supernatant was collected, representing the SDS-soluble fraction (Aβ-SDS). The resultant pellet was then extracted in 70% formic acid (FA) and centrifuged, and the resultant supernatant was collected, representing the SDS-insoluble fraction (Aβ-FA). Before ELISA assay, formic acid extracts were neutralized by 1:20 dilution into 1M Tris phosphate buffer, PH11, and then diluted in sample buffer. Concentrations of Aβ40 and Aβ42 in brain extract and serum were quantitatively measured by ELISA according to the manufacturer’s instructions (Cat. #EZBRAIN40and Cat. #EZBRAIN42, Millipore). Levels of total Aβ come from the sum of Aβ 40 and Aβ 42 of the different fraction.
Quantification of TNF-α, IL-1β and IFN-γ in the Mouse Plasma
TNF-α, IL-1β, and IFN-γ in the plasma of mice were measured using ELISA kits (Cat No.BMS607/2, BMS6002, BMS606, eBioscience, San Diego, CA, USA) as per manufacturer’s instructions.
Toxicity Assessment
The Clinical Laboratory of First Affiliated Hospital of Kunming Medical University was responsible for measuring total bilirubin, alanine aminotransferase (ALT) and aspartate aminotransferase (AST) in the plasma.
Statistical Analysis
Statistical comparisons between groups were assayed using one-way ANOVA measure significant effects of factors. If significant, post hoc testing was done with Tukey’s HSD method, and appropriate p values are reported based on adjustment according to Levene’s test for equality of the variance. A P value of less than 0.05 was considered to be significant. SPSS 19.0 (IBM, Armonk, NY) software was used to perform statistical analysis. The data in the figures are expressed as mean ± SEM.
Results
Tolerability
During treatment period, animal viability, body weight, general activities and daily food consumption were monitored. But we did not find any difference between APP/PS1 transgenic mice and wild-type mice (P>0.05, Fig.2a and Fig.2b). We also detected the serum levels of bilirubin, aminotransferase (AST) and alanine aminotransferase aspartate (ALT) (Fig.2c, the data of bilirubin was not shown because the level was below detection) for all groups, but also no differences between the two treatment groups and control group (P>0.05, APP/PS1 transgenic mice versus wild-type mice fed with control diet). Overall, ginkgetin and curcumin appeared to be well tolerated by APP/PS1 transgenic mice.
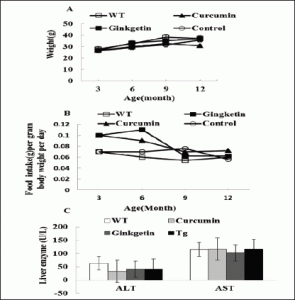
Figure 2. Ginkgetin is well tolerated in APP/PS1 transgenic mice. A: Body weight was measured at 3, 6, 9 and 12 months of age, no significant differences were observed among the 4 groups( P>0.05 versus APP/PS1 transgenic mice fed with control diet). B: Food intake was monitored at 3, 6, 9 and 12 months of age (P>0.05 versus APP/PS1 transgenic mice fed with control diet). C: Liver functional indices such as blood AST and ALT (P>0.05 versus APP/PS1 transgenic mice fed with control diet)
Effects of Ginkgetin on the Brain and Aβ Serum Levels and Deposition in APP/PS1 Transgenic Mice
The effect of ginkgetin on amyloid protein level was assessed in the brain and the serum, the levels of both Aβ40 and Aβ42 in brain homogenates and plasma were analysed by specific sandwich ELISA. Aβ in the SDS fraction represents soluble fraction and the diffuse Aβ plaques, and Aβ in the formic acid fraction represents the fibrillar Aβ plaques. Total Aβ level come from the sum of Aβ 40 and Aβ 42 of the different fraction.
There was no statistically difference in the level of total Aβ and soluble Aβ in 2% SDS in the brain of APP/PS1 transgenic mice treatment with ginkgetin diet and mice fed with normal control diet (ANOVA F=4.360, P>0.05 and F=1.509, P>0.05; Fig.3a), But Aβ level in the formic acid extracts of insoluble pellet was significantly reduced by 52% (ANOVA F= 5.656, P<0.05; Fig.3b,c).and the total Aβ level in the plasma was also obviously lower 59% compared to AD mice fed with standard diet (ANOVA F=4.235, P<0.05; Fig. 3d).
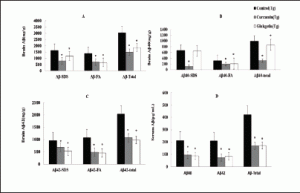
Figure 3. Effects of ginkgetin consumption on Aβ levels in the brain and serum of APP/PS1 transgenic mice. The concentration of Aβ in the brain and serum of each animal was measured by ELISA kit. A: Comparison of total Aβ, Aβ in SDS fraction (Aβ-SDS) and Aβ in formic acid fraction (Aβ-FA) among groups. B: Comparison of total Aβ40, Aβ40-SDS and Aβ40-FA.C: Comparison of total Aβ42, Aβ42-SDS and Aβ42-FA. D: Comparison of total Aβ, Aβ40 and Aβ42 in serum, ginkgetin significantly reduced the Aβ level in the serum (173±18.1 vs 423±19, P<0.05) compared with transgenic mice fed with control diet
Amyloid plaques on coronal sections of the neocortical and hippocampal were detected with immunohistochemical staining. The total Aβ plaques detected by immunohistochemistry were reduced by 51% in the ginkgetin diet group and 55% in the curcumin diet group compared to control group (Tg), the result showed ginkgetin diet obviously reduced Aβ deposits on AD mice brain (ANOVA F=3.351, P<0.05; Fig4).This result was in agreement with the plasma Aβ levels of ELISA analysis.
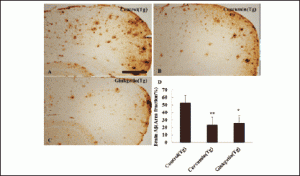
Figure 4. Effects of ginkgetin diet on Aβ deposits in the brain of APP/PS1 transgenic mice. A: Aβ plaques of in neocortex of APP/PS1transgenic mice fed with control diets; B: Fed with ginkgetin; C: Fed with curcumin; D: Comparison of Aβ plaque area fraction in neocortex among groups, Statistical analysis showed significant decreases in the number of plaques in the brain treated with ginkegetin diets. (26±6 vs 51±10, P<0.05) compared with APP/PS1 transgenic mice fed with control diet
Preventive AD-type Pathology Effects of Ginkgetin in APP/PS1 Transgenic Mice
The microgliosis and astrocytosis in the neocortical and hippocampal regions were investigated by immunohistochemistry, reactive astrocytosis was significant reduced by 50% in the brain of ginkgetin diet treatment group and 60% in the curcumin diet treatment group when comparing to the control group fed with normal diet (ANOVA F=8.558, P<0.05; Fig5), but no differences were observed in reactive microgliosis among groups (ANOVA F=4.881, P>0.05).
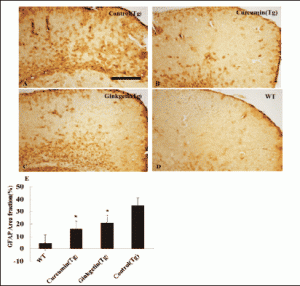
Figure 5. Effects of ginkgetin diet on astrogliosis in the brain. A: Astrogliosis in hippocampus and neocortex of APP/PS1transgenic mice fed with control, B: Transgenic mouse fed with curcumin diet; C: Transgenic mouse fed with ginkgetin diet; D: Wild type mouse fed with control diets; E: Comparison of GFAP area fraction in neocortex and hippocampus among groups. Statistical analysis showed a significantly decrease in the astrogliosis in the brain treated with ginkgetin diets (17±5.5vs.35±5, P<0.05) compared with APP/PS1 transgenic mice fed with control diet
Microhemorrhage (MH) is linked with Aβ deposition in AD and cerebral amyloid angiopathy (CAA) (18) . Here, we detected cerebral MH in brain sections following the previous method (19). After long-term treatment, microhemorrhage rates in the brain of mice fed with ginkgetin food were significantly decreased 69% compared to AD control group fed with normal diet (ANOVA F=2.437, P<0.05; Fig6), but we did not observe change of microhemorrhage rates in curcumin treatment group. Ginkgetin appeared good effect on cerebral amyloid angiopathy.
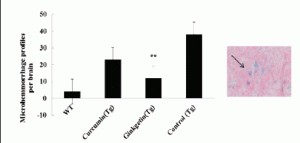
Figure 6. Effects of ginkgetin diet on microhemorrhage profiles. Microhemorrhage events in the form of the number of Prussian blue-positive profiles were counted, and the average number and standard error of hemosiderin deposits was calculated per each brain hemisphere. Statistical analysis showed the mice fed with ginkgetin diet had a significant lower rates of microhaemorrhage (12±3.0 vs 38±7, P<0.05)
Plasma Levels of Inflammatory Cytokines after Treatment
Proinflammatory cytokines, such as TNF-α and IL-1β and IFN-γ could act directly on the neuron and induce apoptosis. Here, we measured levels of the pro-inflammatory cytokines in the serum. Compared with control (37.6 ± 6.1 pg/mL), ginkgetin (17.8 ± 3 pg/mL) diet significant decreased TNF-α level in the plasma of transgenic mice (ANOVA TNF-α, F=2.710, P<0.05), but TNF-α level had no significant change in curcumin treatment group( P>0.05), IL-1and IFN-γ levels in the serum did not appear significant differences among all groups (ANOVA IL-1, F=1.428, P=0.274; IFN-γ, F=0.272, P=0.845; Fig.7).
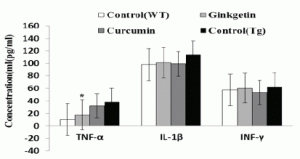
Figure 7. Effects of ginkgetin diet on plasma levels of TNF-a, IL-1β and IFN-γ. Statistical analysis showed the level of TNF-α was significantly decreased in serum of mice (Tg) treated with ginkgetin diet (17.8±3 vs 37.6±6.1, P<0.05) compared with APP/PS1 transgenic mice fed with control diet
Discussion
The results of the present study demonstrate preventive effects of ginkgetin in APP/PS1 transgenic mice without obvious side effects.
According to the amyloid hypothesis, amyloid-β (Aβ) peptide is the major component of senile plaques deposited in the brains of individuals with Alzheimer’s disease (AD) and plays a causative role in the pathogenesis of AD (20). Thus, the important treatment strategy for AD is towards targeting and clearing Aβ. In this study, amyloid-beta levels in plasma and brain of AD transgenic mice appeared very different after ginkgetin treatment. Total Aβ level in the brain had no any change, but plasma Aβ levels significantly decreased and the result appeared be consistent with the Aβ plaque burden. Interestingly, the conflicting data of plasma Aβ levels are present in different AD mouse models, Kawarabayashi et.al (2001) (21) have shown that the plasma levels of Aβ in the Tg2576 mouse model are inversely related to Aβ plaque burden. Unlike the observations by Kawarabayashi et al., DeMattos et al (2002) (22) have reported the plasma Aβ levels did not correlate with the amyloid burden in the brain of the PDAPP mouse model of AD. It is well known, blood Aβ is an attractive biomarker of AD associated with transporters of the blood-brain barrier (BBB), the transport of Aβ between brain and plasma may play a major role in regulating Aβ levels (23). However, plasma Aβ levels would limitedly reflect the status of Aβ abnormality in the central nervous system. Recently, a new study confirmed the significant contribution of BBB to the total clearance of Aβ40 from brain compared to Aβ40 brain degradation (24). It implies a relatively precise Aβ measurement could be performed only in the CSF for the very low protein concentration. To verify this hypothesis, more comparative studied are needed.
One of the features in AD brain is the presence of reactive astrocytes. Astrocytes participate in inflammatory reactions via increased activation of the transcription factor NF-κB (25). NF-κB is one of the primary signaling cascades triggered by TNF-α binding to its receptor (26). In the present research, ginkgetin attenuated the neuroinflammation and appeared to work by decreasing the production of TNF-α and levels of astrogliosis, this evidence supports that NF-κB signalling pathway is crucial a role in the neuroinflammation linked to AD. There is a considerable evidence supports that the role of NF-κB signaling pathway in the AD pathogenesis, it has a capacity to down regulate the activity of proinflammatory transcription factors through their influences of a number of glial and neuronal signaling pathways, such as MAPK cascade (27).
Cerebral amyloid angiopathy (CAA) is a major pathological insult to the neuro vascular unit in AD (28), and is associated with cerebral hemorrhage due to weakening of vascular walls by amyloid deposits and focal inflammation (29). In this research, measurement of Aβ levels in the serum revealed a significant decrease in total amount of Aβ in ginkgetin treated APP/PS1 mice and markedly accompanied by a reduction in microhemorrhages and neuroinflammation. These observations suggest eliminating beta-amyloid deposits and antiinflammation may be effective therapeutic approaches for CAA and parenchymal Aβ deposits.
Taken together, ginkgetin was shown effectively reduced the Aβ levels in the brain and blood, decreased cerebral microhemorrhage, lowed astrogliosis, ameliorated inflammation in APP/PS1 transgenic modle, this is the first observation that ginkgetin ameliorates pathological changes in vivo, but pathophysiology mechanisms of Aβ clearance need further research.
Acknowledgements: This work was supported by grants from MOST (2011CB944200) and National Natural Science Foundation (81441136)
Conflict of interests: The authors do not have any conflict of interest to disclose.
Ethical standards: This experiment complies with current laws of the country in which they were performed.
References
1. Serrano-Pozo, A., M. P. Frosch, E. Masliah, and B. T. Hyman. «Neuropathological Alterations in Alzheimer Disease» Cold Spring Harb Perspect Med 1, no. 1 (Sep 2011): a006189.3.
2. Wirths, O., G. Multhaup, and T. A. Bayer. «A Modified Beta-Amyloid Hypothesis: Intraneuronal Accumulation of the Beta-Amyloid Peptide–the First Step of a Fatal Cascade.» J Neurochem 91, no. 3 (Nov 2004): 513-20
3. Chinese Pharmacopoeia Commission «Pharmacopoeia of the People’s Republic of China» China Medical Science Press, Beijing: 2010.
4. SK, Dash. «Ginkgo Biloba in Alzheimer’s Disease.» Disease. Austin J Clin Neurol 2, no. 2381-9154 (2015): 7.
5. Al-Attar, A. M. «Attenuating Effect of Ginkgo Biloba Leaves Extract on Liver Fibrosis Induced by Thioacetamide in Mice.» J Biomed Biotechnol 2012 (2012): 761450.
6. Smith, J. V., and Y. Luo. «Studies on Molecular Mechanisms of Ginkgo Biloba Extract» ApplMicrobiol Biotechnol 64, no. 4 (May 2004): 465-72.
7. You, O. H., S. H. Kim, B. Kim, E. J. Sohn, H. J. Lee, B. S. Shim, M. Yun, B. M. Kwon, and S. H. Kim.»Ginkgetin Induces Apoptosis Via Activation of Caspase and Inhibition of Survival Genes in Pc-3 Prostate Cancer Cells.» Bioorg Med Chem Lett 23, no. 9 (May 1 2013): 2692-5.
8. Kang, S. S., J. Y. Lee, Y. K. Choi, S. S. Song, J. S. Kim, S. J. Jeon, Y. N. Han, K. H. Son, and B. H. Han.»Neuroprotective Effects of Naturally Occurring Biflavonoids» Bioorg Med Chem Lett 15, no. 15 Aug 1 2005): 3588-91.
9. Birks J, Grimley Evans J. «Ginkgo Biloba for Cognitive Impairment and Dementia «. no. 2 (25 March 2008. 2009):1- 10
10. Perry, E., and M. J. Howes. «Medicinal Plants and Dementia Therapy: Herbal Hopes for Brain Aging?» CNS Neurosci Ther 17, no. 6 (Dec 2011): 683-98
11. Yang, F., G. P. Lim, A. N. Begum, O. J. Ubeda, M. R. Simmons, S. S. Ambegaokar, P. P. Chen, et al.»Curcumin Inhibits Formation of Amyloid Beta Oligomers and Fibrils, Binds Plaques, and Reduces Amyloid in Vivo.» J Biol Chem 280, no. 7 (Feb 18 2005): 5892-901.
12. Jankowsky, J. L., D. J. Fadale, J. Anderson, G. M. Xu, V. Gonzales, N. A. Jenkins, N. G. Copeland, et al. «Mutant Presenilins Specifically Elevate the Levels of the 42 Residue Beta-Amyloid Peptide in Vivo: Evidence for Augmentation of a 42-Specific Gamma Secretase.» Hum Mol Genet 13, no. 2 (Jan 15 2004): 159-70.
13. Zhao, X., Y. Zou, H. Xu, L. Fan, H. Guo, X. Li, G. Li, X. Zhang, and M. Dong. «Gastrodin Protect Primary Cultured Rat Hippocampal Neurons against Amyloid-Beta Peptide-Induced Neurotoxicity Via Erk1/2-Nrf2 Pathway.» Brain Res 1482 (Oct 30 2012): 13-21
14. Yamakoshi, J., M. Saito, S. Kataoka, and M. Kikuchi. «Safety Evaluation of Proanthocyanidin-Rich Extract from Grape Seeds» Food and Chemical Toxicology 40, no. 5 (05/01 2002): 599-607.
15. U.S. Department of Health and Human Services, Food and Drug Administration, Center for Drug Evaluation and Research (CDER)»Guidance for Industry Estimating the Maximum Safe Starting Dose in Initial Clinical Trials for Therapeutics in Adult Healthy Volunteers «. (2005): 1-27
16. Wang, Y. J., A. Pollard, J. H. Zhong, X. Y. Dong, X. B. Wu, H. D. Zhou, and X. F. Zhou. «Intramuscular Delivery of a Single Chain Antibody Gene Reduces Brain Abeta Burden in a Mouse Model of Alzheimer’s Disease.» [In Eng]. Neurobiol Aging 30, no. 3 (Aug 6 2009): 364-76.
17. Wang, Y. J., X. Wang, J. J. Lu, Q. X. Li, C. Y. Gao, X. H. Liu, Y. Sun, et al. «P75ntr Regulates Abeta Deposition by Increasing Abeta Production but Inhibiting Abeta Aggregation with Its Extracellular Domain.» The Journal of Neuroscience: The Official Journal of the Society for Neuroscience 31, no. 6 (02/09 2011): 2292-304.
18. Yates PA, Sirisriro R, Villemagne VL, Farquharson S, Masters CL, Rowe CC; AIBL Research Group «Cerebral Microhemorrhage and Brain Β-Amyloid in Aging and Alzheimer Disease» Neurology 77, no. 1 (2011): 48-54.
19. Wang, Y. J., P. Thomas, J. H. Zhong, F. F. Bi, S. Kosaraju, A. Pollard, M. Fenech, and X. F. Zhou.»Consumption of Grape Seed Extract Prevents Amyloid-Beta Deposition and Attenuates Inflammation in Brain of an Alzheimer’s Disease Mouse.» Neurotoxicity Research 15, no. 1 (01/01 2009): 3-14.
20. Hardy, J., and D. J. Selkoe. «The Amyloid Hypothesis of Alzheimer’s Disease: Progress and Problems on the Road to Therapeutics.» Science 297, no. 5580 (07/19 2002): 353-56.
21. Kawarabayashi T, Younkin LH, Saido TC, Shoji M, Ashe KH, Younkin SG. «Age-Dependent Changes in Brain, Csf, and Plasma Amyloid (Beta) Protein in the Tg2576 Transgenic Mouse Model of Alzheimer’s Disease» J Neurosci 21, no. 2 (2001): 372–81.
22. DeMattos RB, Bales KR, Parsadanian M, O’Dell MA, Foss EM, Paul SM, Holtzman DM. «Plaque associated Disruption of Csf and Plasma Amyloid-Beta (Abeta) Equilibrium in a Mouse Model of Alzheimer’s Disease.» Journal of Neurochemistry 81 (2002): 229-3
23. Cho, S. M., H. V. Kim, S. Lee, H. Y. Kim, W. Kim, T. S. Kim, D. J. Kim, and Y. Kim. «Correlations of Amyloid-Beta Concentrations between Csf and Plasma in Acute Alzheimer Mouse Model» Sci Rep 4 (2014): 1-4.
24. Qosa, H., B. S. Abuasal, I. A. Romero, B. Weksler, P. O. Couraud, J. N. Keller, and A. Kaddoumi. «Differences in Amyloid-Beta Clearance across Mouse and Human Blood-Brain Barrier Models: Kinetic Analysis and Mechanistic Modeling.» Neuropharmacology 79 (Apr 2014): 668-78.
25. Spilsbury, A., D. Vauzour, J. P. Spencer, and M. Rattray. «Regulation of Nf-Kappab Activity in Astrocytes: Effects of Flavonoids at Dietary-Relevant Concentrations.» Biochem Biophys Res Commun 418, no. 3 (Feb 17 2012): 578-83.
26. Ramasamy, R., S. F. Yan, and A. M. Schmidt. «Receptor for Age (Rage): Signaling Mechanisms in the Pathogenesis of Diabetes and Its Complications.» Ann N Y Acad Sci 1243 (Dec 2011):88-102.
27. Gonzalez-Gallego, J., M. V. Garcia-Mediavilla, S. Sanchez-Campos, and M. J. Tunon. «Fruit Polyphenols, Immunity and Inflammation.» Br J Nutr 104 Suppl 3 (Oct 2010): S15-27.
28. Bell, R. D., and B. V. Zlokovic.»Neurovascular Mechanisms and Blood-Brain Barrier Disorder in Alzheimer’s Disease» Acta Neuropathol 118, no. 1 (Jul 2009): 103-13
29. Yang, J., Y. Ji, P. Mehta, K. A. Bates, Y. Sun, and T. Wisniewski. «Blocking the Apolipoprotein E/Amyloid-Beta Interaction Reduces Fibrillar Vascular Amyloid Deposition and Cerebral Microhemorrhages in Tgswdi Mice.» J Alzheimers Dis 24, no. 2 (2011): 269-85.